Nitrogen is essential for plants to grow.
But while nitrogen makes up 78% of the air in the Earth’s atmosphere, it is inert and unusable to plants in that form. Legumes (such as peas, beans and alfalfa) are the only crops able to team with bacteria in their roots to chemically convert nitrogen from the air into a form that living organisms can use — something other crops cannot do well, if at all.
Legumes make nodules in their roots for the bacteria to live in. The bacteria convert the nitrogen and feed it to the plant. In return, the plant feeds the bacteria carbon.
In an effort to understand how legumes “fix” nitrogen from the air so it can be used by the plant, a team of Clemson University researchers have conducted National Science Foundation-funded research to explore the expression of genes during that nodulation process in the model plant Medicago truncatula, an alfalfa that grows in lawns and fields.
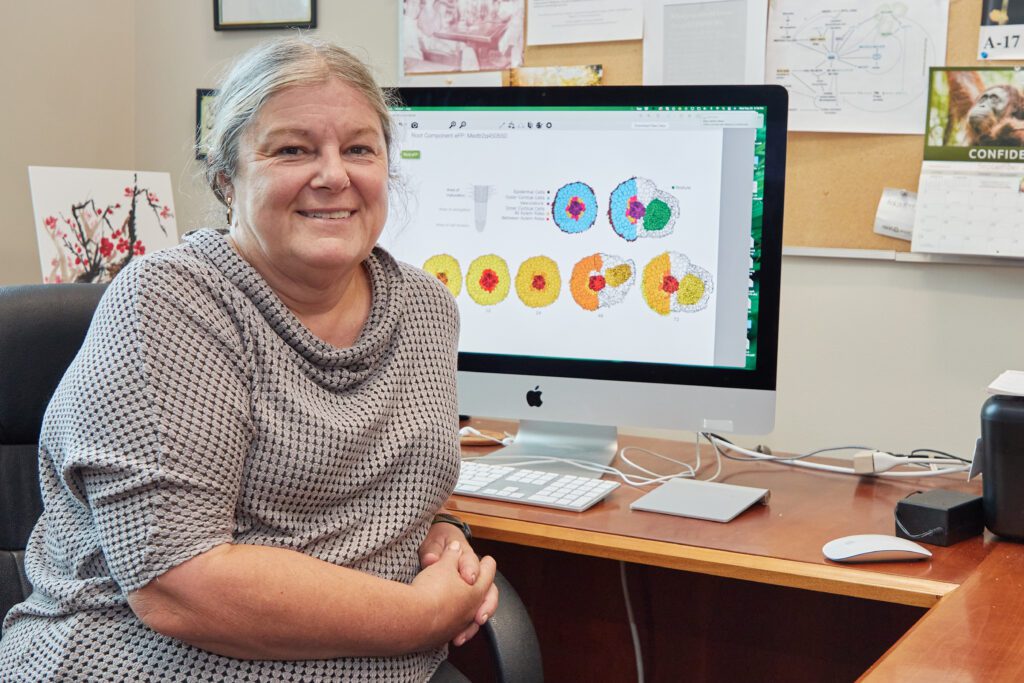
Reducing the need for fertilizer
Scientists say understanding the process could make it possible to activate it in other plants that cannot make root nodules but could support the right bacteria. That could increase sustainable crop production, save farmers money and help rid the planet of a pollutant that is damaging the world’s waters. Only about half the nitrogen fertilizer added to fields is actually taken up by crops. The rest enters the water table, polluting wells and running off into rivers, lakes and oceans.
“The process of finding out what a gene interacts with and where it is expressed can be a real slow slog if you’re doing it one gene at a time,” said Julia Frugoli, Alumni Distinguished Professor in the Department of Genetics and Biochemistry, and one of the lead researchers on the project.
There are 54,893 genes in each M. truncatula cell.
“We took a transcriptomics approach where we looked at all of the genes in a very defined process that took out as many variables as we could about how this interaction starts, how the bacteria signals to the plant and how the plant signals to the bacteria. And we looked at the genes at five fixed time points.”
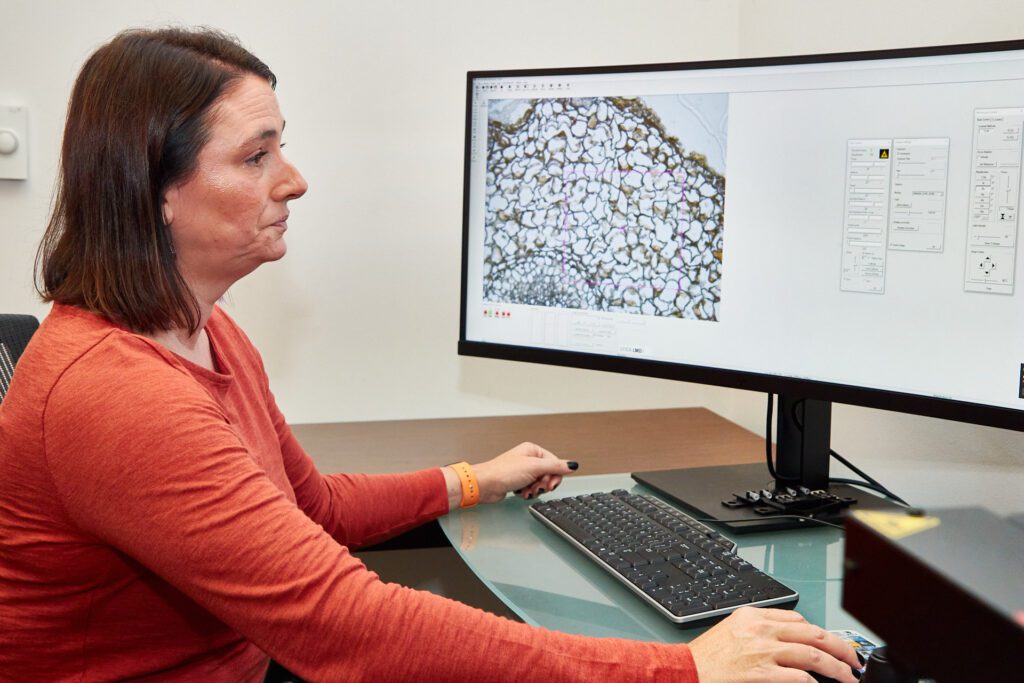
High-tech tools
Using laser capture microdissection through the Clemson Light Imaging Facility (CLIF) and high-performance computing through the Palmetto supercomputer at Clemson, the researchers were able to measure gene expression — the information stored in DNA that dictates the production of proteins.
That data is now publicly available through an online interactive database hosted by the University of Toronto.
“Nobody else will have to do this experiment to find out if their gene is involved,” Frugoli said. “That was a big deal because I think we tied up the laser microscope at CLIF for close to three years every day cutting tissue. It was a massive undertaking.”
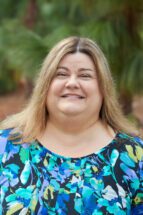
Laser capture microscopy allows the researchers to take a thin slice of tissue and place it on a membrane that has a low melting temperature. Researchers then use a stylus on a computer screen to trace around the area of cells they want to examine. The computer transfers the information to the laser, which cuts that part out, melting the polyethylene naphthalate (PEN) membrane as it does so to separate it from the main tissue. The portion of the tissue to be studied drops into a tube or some other type of container that can then be used for work downstream such as RNA sequencing and then bioinformatics.
“This allows you to very specifically isolate that area of tissue that you want, in this case just a few cells,” said Terri Bruce, a research assistant professor in the Department of Bioengineering and CLIF facility director.
But it’s not enough to just cut a few cells, said Rhonda Powell, research lab manager at CLIF.
“You cut those same few cells on section after section after section,” she said.
The researchers harvested cross sections of the roots at five timepoints — at 0-, 12-, 24-, 48- and 72-hours post inoculation.
The RNA extracted from the individual cells was turned into deoxyribonucleic acid (DNA), which was decoded using modern DNA sequencers and then analyzed on the Palmetto supercomputer with algorithms developed by Alex Feltus, a professor in the Department of Genetics and Biochemistry, and members of his lab.
“Before we had advanced technology like lasers, high throughput DNA sequencers and supercomputers, you would study one or two genes at a time. Now, you can take a look at all genes acting in concert in a biological process like root nodulation,” Feltus said.
Frugoli said about 40 years ago, the thought was that if we could find the genes that allow legumes to get nitrogen out of the air, those genes could be moved to another plant and enable them to do the same thing. However, as science has gotten better, researchers discovered most of these genes were already in all the other plants that don’t fix nitrogen.
“It’s how they’re regulated, so now it’s all about how that regulation — why does this gene come on when my plant (M. truncatula) encounters rhizobia (the bacteria), but it doesn’t happen when wheat is in the soil with rhizobia. Wheat doesn’t even recognize the rhizobia,” she said.
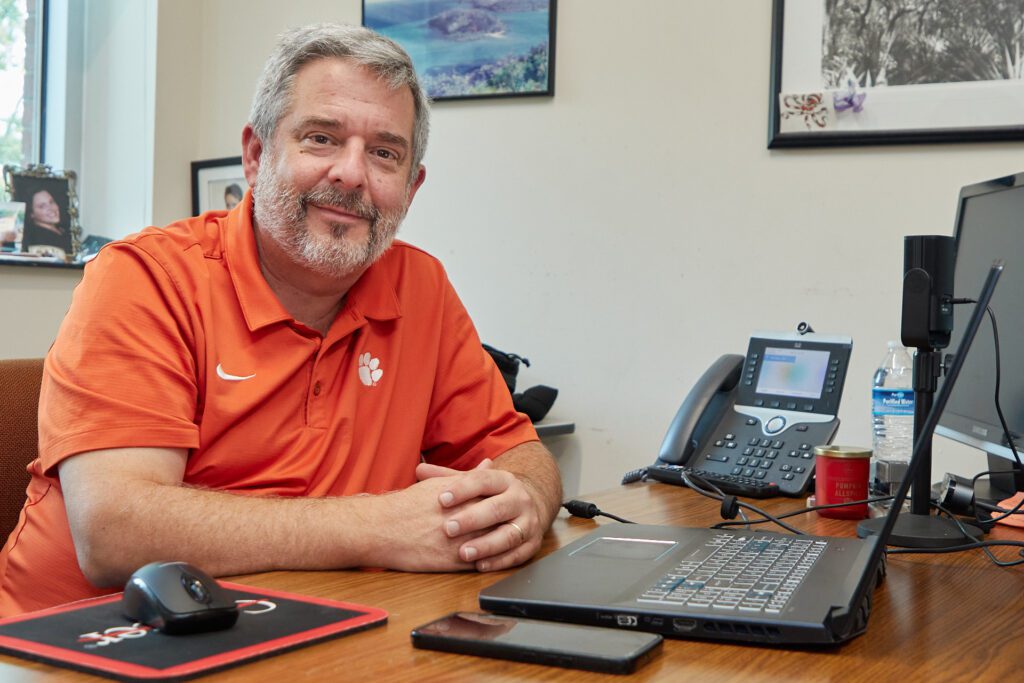
Some plants make nodules but don’t have the bacteria living inside those bumps. Even if you get bacteria living inside the bumps, they still have to pass the nitrogen to the plant.
“All of these stages have some regulation that is not completely present in other systems,” she said.
Feltus said, “The complex regulation of 50,000 genes is something you couldn’t see if you didn’t look at all 50,000 genes at one time. If you look at one at a time, you wouldn’t see it.”
One step toward the ultimate goal
Having the data publicly accessible online is a step toward the ultimate goal of getting plants to make their own fertilizer, Feltus said.
“It is progress toward that goal that would have massive impacts on our planet,” he said. “This is exactly what Thomas Green Clemson wanted, but we’re using lasers and supercomputers to do it.”
Thomas Green Clemson was a longtime advocate for an agricultural college in the Upstate, saying he believed no permanent improvement in agriculture could come without a knowledge of the pertinent science. He left his home and fortune to the state of South Carolina to create the institution that now bears his name.
“We need more of this high-tech applied to complex problems like ensuring food security to 8 billion people while [the] climate changes,” Feltus said.
Publication of the paper titled, “Laser capture microdissection transcriptomes reveals spatiotemporal tissue gene expression patterns of M. truncatula roots responding to rhizobia” in the journal Molecular Plant-Microbe Interactions marks the conclusion of the research done through the NSF grant IOS #1444461.
The RNA-seq data generated by a team that included lead author Elise Schnabel, now a research associate with the Clemson Department of Plant and Environmental Sciences, was shared with the Provart Lab at the University of Toronto, which developed a series of electronic interfaces to visualize data from various plants, including M. truncatula. The data is available in the Medicago ePlant at https://bar.utoronto.ca/eplant_medicago/.
Other Clemson scientists involved in the project were Jacklyn Thomas, Rabia El-Hawaz, Yuevao Gao, William Poehlman and Suchitra Chavan.
Get in touch and we will connect you with the author or another expert.
Or email us at news@clemson.edu