A Clemson University scientist’s research could lay the foundation for new therapeutics for diabetes insipidus, a rare disorder characterized by intense thirst and the excretion of large amounts of urine.
David Jacobson, an assistant professor in the Department of Chemistry, is studying the structural dynamics of human arginine vasopressin receptor 2 (AVPR2) — a membrane protein that is expressed in human kidneys and involved in the production of urine — and how those dynamics are changed by disease-causing mutations.
The research is funded through a National Institutes of Health K99/R00 Pathway to Independence Award he received in 2021 when he was a post-doctoral fellow at the University of Colorado. The five-year competitive grant provides promising post-doctoral scientists up to two years of mentored support followed by up to three years of support as an independent investigator. In the last fiscal year, only 419 such awards were made nationwide across all areas of biomedical research. Jacobson is the first faculty member to bring this award to Clemson University for the independent phase. Clemson’s first postdoctoral K99/R00 awardee came last year when Cherice Hill from the Department of Bioengineering received an award.
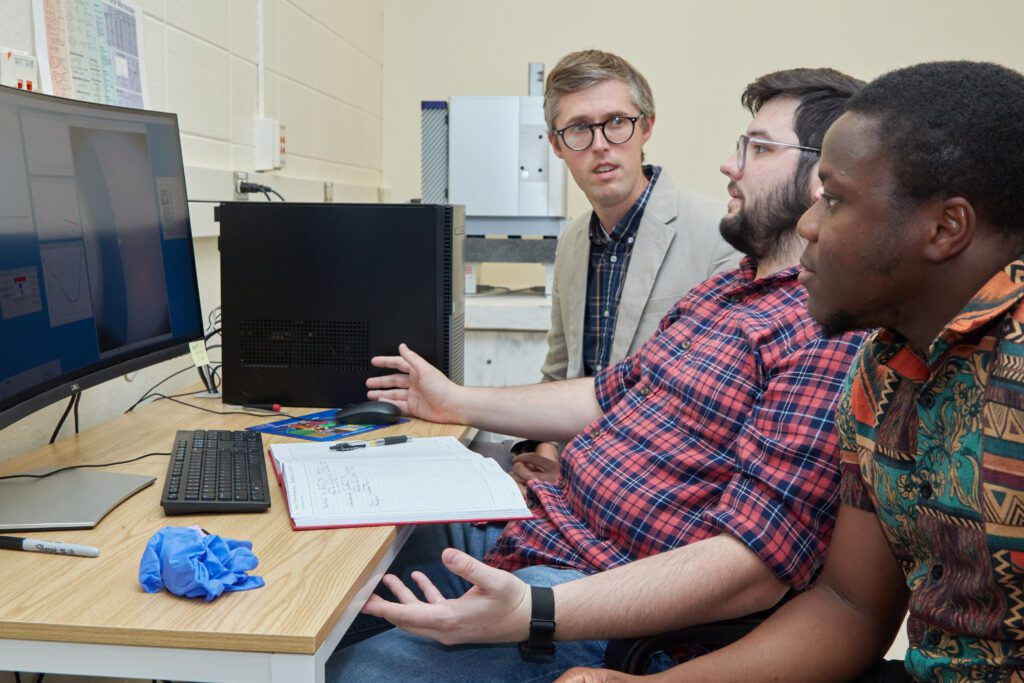
Disease-causing mutation
There are more than 90 known single amino acid mutations in AVPR2 that cause diabetes insipidus, a rare disorder that causes the body to make too much urine. While most people make 1 to 3 quarts of urine a day, people with diabetes insipidus can produce up to 20 quarts of urine a day, according to the National Institutes of Health. Diabetes insipidus is rare, affecting about 1 in 25,000 people worldwide.
Diabetes insipidus is not the same as the more commonly known diabetes mellitus, a disease characterized by sustained high blood sugar levels.
“The diseases are not really related, and it’s kind of a historical accident that they have the same name. They both involve some abnormality in the urine, but the underlying mechanisms are totally different, and they involve different organs,” Jacobson said.
Difficult to study
Membrane proteins are difficult to study by traditional biochemical methods because they are not water soluble. About 30% of all proteins are membrane proteins.
“Membrane proteins are the targets of about 50% of current Food and Drug Administration approved drugs, the reason being that because they are in the cell membrane, they interact with the inside of the cell as well as the extracellular environment where a drug could be supplied,” Jacobson said.
Jacobson’s lab is studying how disease-causing mutations affect the stability and energetics of the individual proteins.
Proteins are made of long strings of amino acids folded into three-dimensional shapes. By folding into distinct shapes, proteins can perform different tasks despite being composed of the same building blocks.
To measure the stability of a protein, researchers need to be able to unfold it and refold it.
Traditionally, that’s done by adding some type of denaturing chemical to cause the protein to unfold. When the denaturant is removed, the protein folds again. But membrane proteins tend to not refold after they’ve been unfolded, Jacobson said.
Using force
So, Jacobson uses an atomic force microscope to exert mechanical force to unfold a part of an individual molecule.
“One of the beauties of the single molecule measurements is because we’re pulling from a specific point on the protein, we don’t have to unfold the whole thing. We can pull on a little bit and unfold part of it, and then lower the force and get it to refold,” he said.
By understanding the mechanism by which a mutation leads to disease at the molecular level, researchers could, in the future, potentially design strategies to overcome the negative effect of the mutation, Jacobson said.
“For example, if the mutation is destabilizing a particular part of the protein, you could design a binding partner that stabilizes that part of the protein,” he said. “Drugs act on proteins at the molecular level. So, to think rationally about drugging strategies, you need to understand molecularly what’s going on with the protein that would be the target of the drug.”
Get in touch and we will connect you with the author or another expert.
Or email us at news@clemson.edu